For access to the IAT membership list and discussion
areas, click on these links
Current
IAT status and membership
Discussion
Forum
A Workshop on
Liquids and Disordered Materials Science at
the Spallation Neutron Source
16-17 October 1998
Intense Pulsed Neutron Source, Argonne National
Laboratory
Co-sponsored by Argonne and Oak Ridge National
Laboratories
Co-chairs D. L. Price (ANL) and B. K. Annis
(ORNL)
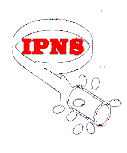
Compiled by T. Habenschuss (ORNL)
4 Nov 1998, Version 1.0
Table of Contents
Introduction
Why neutrons?
Impact of increased flux at the SNS on liquids
and disordered material science
Potential liquid and disordered materials research
to be conducted at the SNS
Scientific case for a liquid/amorphous diffractometer
- overview
Liquid/amorphous diffractometer specification
Other instruments
Appendix A. Current And Potential Research to
Be Conducted at the SNS by the Liquids and Disordered Materials Science
Community
Appendix B. Organizing Committee
Appendix C. Workshop Agenda
Appendix D. List of Workshop Participants
Acknowledgments
Introduction
(Back)
The construction of a 1MW pulsed neutron source,
the Spallation Neutron Source (SNS), at Oak Ridge National Laboratory,
provides a unique opportunity to advance the science of liquids and disordered
materials in the US. The purpose of this report is to survey the new research
that will be enabled by this world class facility, and to lay out the instrumental
needs of the scientific community engaged in the study of liquids and disordered
materials. To this end a Disordered Materials Workshop, sponsored by ORNL
and ANL, was held on 16-17 October 1998. This document is the outgrowth
of this workshop.
The field of liquids and disordered materials
ranges widely from low density fluids (supercritical state), through liquids,
melts, solutions, glasses, amorphous materials, to semicrystalline solids.
The materials of interest can be characterized by some degree of ordering
short of the perfect crystalline state. It is precisely the short to intermediate
range order that is the focus of this field of science, both of fundamental
interest and from a practical point of view. The understanding of the structure
and dynamics of non-crystalline materials has lagged behind that of crystalline
materials due to the limited order encountered in the former. Yet many
of the materials of technological utility depend on the nature of disorder
found in these materials - for example, polymers, amorphous semiconductors,
chemical process systems, oil recovery, supercritical solvents in "green
chemistry" applications, etc.
The materials of interest may be in the
form of bulk samples, films, fibers, and materials in confined environments.
The length scales covered include local, short range atomic/molecular order
on the few Ångstroms scale, intermediate range order (intermolecular
correlations, networks in glasses, etc), to mesoscopic dimensions of 10's
to 100's of nanometers (surfactant ordering, protein folding, liquid crystals,
etc.). The dynamics of interest range from local, atomic/molecular motions
to collective phenomena in, for example, glass transitions. Parameter space
includes temperature, pressure, magnetic and electric fields, and dynamic
conditions (flow, shear, pulsed deformation).
The two primary areas of experimental research
are the structure and dynamics of disordered materials. In this report
we focus on those experimental techniques where neutrons provide unique
advantages over other structural and spectroscopic tools. We emphasize
those studies which can not be done with present facilities and will benefit
from the large increase in power represented by the SNS. The community
employs neutron scattering techniques (liquid/amorphous diffractometers,
including anomalous scattering and polarization analysis), spectrometers
(inelastic, quasi-elastic), SANS, reflectometry, and texture measurements.
Except for the liquid/amorphous diffractometer, these techniques are being
addressed by other focus groups for the SNS, and we will only briefly outline
our needs from the liquid and disordered materials perspective. Appropriate
input will be given to the focus groups concerned with specifying these
instruments. However, the liquid/amorphous diffractometer is unique
to this community, and we will focus the report on its specification.
In what follows we will list the characteristics of neutrons and the impact
of increased flux from the SNS; state the scientific opportunities in liquids
and disordered materials science; give a detailed specification of a liquid/glass
diffractometer (L&AD), and the needs of the community for other experimental
beamlines.
Why neutrons?
(Back)
There are a number of advantages to neutrons
as a probe of materials[1]. Some of these are unique, while others complement
experimental techniques such as X-ray scattering.
-
energies
of thermal neutrons matched to atomic motions
-
from neV of polymer reptation, to molecular
vibrations and lattice modes, to eV transitions within electronic structures
of materials
-
wavelengths
of thermal neutrons matched to atomic/molecular
dimensions
-
structural info from 10-5 to 104Å
length scales (wave function of hydrogen to macromolecules)
-
neutrons see nuclei,
complementing X-rays, which see electron clouds
-
neutrons sensitive to atomic correlations
directly
-
scattering cross section not a monotonic function
of atomic number
-
see light atoms (H, Li, N, O, etc.)
-
possibility of distinguishing neighboring
elements
-
cross section varies among isotopes
-
isotopic substitution (separate out correlations)
-
contrast variation (highlight or eliminate
parts of system)
-
cross section independent of momentum transfer,
Q
-
TOF scattering experiment gives data up to
very high momentum transfer, Q
-
neutron's magnetic
moment suited to study of
-
magnetic structures and magnetic fluctuations
in materials
-
neutrons perturb
experimental systems only weakly
-
obtain atom-atom correlations directly
-
neutrons are highly
penetrating and non-destructive
-
probe complex, delicate biological materials;
where X-rays might damage samples
-
a bulk probe, where X-rays might only probe
the surface
-
investigate interior of materials; examine
real industrial products
-
materials containing heavy elements which
might not be transparent to x-rays
-
penetrate through complex environments (furnaces,
cryostats, pressure cells)
-
neutrons allow application of anomalous
dispersion
-
selected nuclei with resonances can be used
to highlight correlations
Impact of increased flux
at the SNS on liquids and disordered material science.
(Back)
The quantum jump in flux available at the
SNS over existing neutron sources will result in higher
resolution in space and time, measurements of weaker
signals, over shorter time,
on smaller systems, and in more extreme
sample environments [1]. Of course, these impacts apply to all
areas of neutron science.
-
higher resolution
in space and time
-
accurate and precise structure refinements
-
excitation spectra of complex systems
-
dynamics of quasicrystals
-
precise shapes of potential functions
-
spectral studies of binding of molecules (chromophores,
therapeutic biomolecules)
-
excited state distortions of dissolved molecules
in solution environments
-
measure weaker signals
-
smaller differences can be measured
-
isotopic substitution studies; smaller differences
in isotope cross-sections feasible (Carbon: molecular biology, biotechnology,
pharmaceuticals, polymers)
-
polarization analysis (separate coherent and
incoherent signal)
-
full spherical polarization analysis methods
in magnetism
-
subtle effects and small changes (high T superconductors)
-
measurements over shorter
time
-
wider parameter space (T, P, electric and
magnetic field, concentrations, pH, etc)
-
solve problems rather than single experiments
-
increase experimental throughput
-
real-time studies:
-
structure and dynamics of reaction species
-
kinetic processes and material behavior under
non-equilibrium conditions
-
unit cost reduction - routine industrial exploitation
-
measurements on smaller
systems
-
inherently small (crack tip strain distribution)
-
only small amounts are available (new materials,
isotopes)
-
probe spatial variation
-
structure and dynamics of surfaces and interfaces,
thin layers
-
lower concentrations (biomolecular concentration
regimes)
-
more extreme sample
environments
-
only small sample volumes possible (diamond
anvil cell)
-
environmental time is short (extremely low
temperatures)
Potential liquid and disordered
materials research to be conducted at the SNS
(Back)
Examples of the structural research that might
be tackled on the new spallation source can be divided into those requiring
maximum flux and Q range, but modest resolution (mostly liquid materials),
and those involving solid (glassy, amorphous or crystalline) materials,
which require improved resolution to resolve details of the local structure.
Traditionally, advances in this field have occurred whenever the available
neutron fluxes are significantly enhanced, (e.g. when ILL started operation
and then again when ISIS started operation). In all cases the examples
represent extensions of work being carried out or planned at existing facilities:
the advantages of the improved flux and/or resolution at the SNS mean that
in every case these fields will progress to more complex materials and
advanced sample environments, with particular emphasis on determining modifications
to the structure induced by changes in the chemical or isotopic composition,
temperature, pressure, or physical state of materials. (If carbon isotope
substitution can be used, this would open up large areas of organic, macromolecular
and biological systems).
Similarly, the increased flux from the
new spallation source will impact the study of dynamics in such areas as
vibrational motion in network inorganic glasses, polymers and soluble proteins,
ion mobility in super-ionic glasses and conducting polymers, relaxation
in bulk and interfacial water, boson peaks, and coherent inelastic process
such as Brillouin scattering.
What follows is a partial list of topics
which are currently of interest to the liquids and disordered materials
community. Appendix A lists selected, short descriptions of specific research
topics that are to be addressed at the SNS.
-
Fundamental fluids
-
quantum fluids - 3He, 4He,
mixtures
-
liquid hydrogen
-
Molecular fluids and solutions
-
hydrogenous liquids
-
liquids under extremes of temperature and
pressure
-
compare to simulation; map out T/P parameter
space
-
supercritical solutions for environmentally
benign chemistry
-
non-aqueous electrolyte solutions
-
Water and aqueous solutions
-
especially at extreme T/P conditions - supercooled,
supercritical
-
structural and dynamic similarities between
supercooled and interfacial water
-
Brillouin spectroscopy of bulk and interfacial
water
-
Fluids in confined geometries
-
liquids confined in, for example, Vycor or
selfassembled mesoscopic materials
-
fluid - membrane interactions
-
Liquid and amorphous polymers and polymer
blends
-
local structure and motion, and effects of
ions in electrolytes
-
polymer structure and dynamics under shear
-
intermolecular structure of polyolefins and
blends; phase separation/miscibility
-
local structure and its effect on electronic
properties, including conducting polymers
-
orientation in polymer fibers
-
Amorphous fraction in semi-crystalline polymers
-
mesophase transformations in semicrystalline
polymers: conformational disorder
-
Crystalline/liquid hybrid materials
-
Intercalated liquids in e.g. graphite, clays
-
liquid crystals
-
Glasses, fundamental
-
definition of basic structural units in glasses
and the effect of modifiers on the distribution of bond lengths and angles
-
effect of superstructural units on vibrational
density of states in inorganic network glasses
-
thermodynamic fluctuations at the glass transition
-
fluctuations in chemical order in multicomponent
glasses
-
the crystallization process, pre-melting effects
-
crystallization in production of glass ceramics
-
orientational order in glass fibers
-
nature of the boson peak in glasses as a f(T,P)
-
understand the nature of "fragile" and "strong"
glasses
-
Hybrid glasses
-
mixed oxides, polymer/inorganic glasses
-
Fast ion conducting glasses
-
Searching phase diagrams for the best electronic
performance
-
in situ study of battery materials e.g. charging/discharging
-
thin films in electric fields
-
use of QENS to investigate the mobility of
conducting ions
-
Semicrystalline materials
-
crystalline materials with significant disorder
-
non-crystalline materials with significant
local order, e.g. graphite, clay-type materials, network silicas
-
electronic processes in covalent systems such
as oxides; includes ferroelectrics, metal/insulator transition, superconductivity
and spin-lattice coupling
-
Geologically relevant materials
-
liquids and glasses of mixtures/solutions
of lanthanide, actinide, alkali and alkaline earth silicates, phosphates
and borates
-
amorphisation of geological materials under
extreme pressure
-
Biologically relevant fluids
-
hydration and conformation in organic materials
of biological interest
-
polyelectrolyte liquids
-
location and interaction of biologically significant
ions, e.g. Ca, Mg ions
-
temperature dependence of biological fluids
and solutions
-
dynamics of caged or interfacial water in
proteins, DNA, and membranes
-
vibrational spectroscopy of surface residues
on soluble globular proteins
-
Metals and metallic alloys, semiconductors
-
liquid metals and alloys
-
metallic (amorphous) glasses
-
diffuse scattering from chemical and positional
disorder in alloys
-
amorphous silicon fuel cells
-
Molten salts
-
molten carbonates for fuel cells
Scientific case for a
liquid/amorphous diffractometer (L&AD)- overview
(Back)
Currently the only dedicated facility within
the US for neutron diffraction studies of the non-crystalline states of
matter at the atomic length scale is GLAD at the IPNS pulsed neutron source,
and the new source would provide an advancement in this capability by a
factor of about 100. The factor 6 or more enhancement in flux compared
to the existing ISIS source or the (upgraded) LANSCE source, will allow
the possibility of constructing either a diffractometer whose resolution
is equivalent to existing facilities, such as HIPPO at LANSCE, SANDALS
at ISIS, or the D4B diffractometer at ILL, but whose neutron flux is dramatically
higher
than at those facilities, or else a diffractometer whose count rate is
similar to the existing facilities, but whose resolution is double
that currently available anywhere in the world for this kind of work. The
latter instrument would be equivalent in resolution to the new GEM diffractometer
currently under construction at ISIS, but with at least 6 times the count
rate. The instrument specification outlined below allows for both possibilities,
subject only to budget and engineering constraints. If budget restrictions
prevent full realization of the concept described then the community would
opt for the high count-rate, modest resolution option in the first instance,
with adoption of the higher resolution option at a later stage.
The scientific case for a disordered
materials diffractometer at the SNS derives from the fact that the
US currently has NO world-class facility for studying the local atomic
ordering in materials with neutrons.
Experience in Europe and Japan has shown
that such a diffractometer is likely to be oversubscribed by a factor of
2 - 2.5. In addition, provided the correct infrastructure for gaining access
to the facility is set up, that experience indicates that the User base
will grow and evolve as new groups of materials scientists, chemists, geologists
and biologists realize the new opportunities for research that the diffractometer
will provide.
The field of diffraction from disordered
matter has stringent requirements for detector stability and uniformity,
detector shielding, beamline collimation, wave vector transfer (Q) range
(with appropriate resolution in each range), and neutron flux in all Q-ranges.
Such a specification has never been realized by any existing or proposed
powder diffractometer used for crystallographic studies, and it is essential
that a diffractometer dedicated to local structure studies should be a
separate instrument at the SNS. User demand is likely to require this in
any case.
Liquid/amorphous diffractometer
specification (Back)
Experience at existing pulsed and reactor
neutron sources gives very clear indications for the specification of a
disordered material diffractometer at the SNS. A singular departure from
existing facilities of this kind is the requirement for a very large range
of Q values for the new instrument: typically 0.02Å-1
to 80Å-1 was felt to be the minimum that would be acceptable
but still achievable with careful design. The specified minimum Q is an
order of magnitude smaller than existing liquid & amorphous diffractometers,
and is required to accommodate the increasingly complex materials that
are being investigated by this method. This range should be enhanced further
if the means could be found, possibly by inserting converging collimators
in front of the sample. It was felt to be especially important that at
low Q values there should be at least a decade of overlap with any SANS
facility that was developed at SNS, to ensure that diffraction data on
any materials that would require both L&AD diffractometer and SANS
instruments could be satisfactorily merged.
Equally the maximum resolution for the
research glasses and semicrystalline solids (about 0.25% in backscattering)
would involve an unacceptable loss in count rate (about a factor of 6)
for the research on liquids, where a more modest resolution (around 0.5%
in backscattering) was needed. These different requirements could potentially
be met in a single diffractometer, with two sample tank positions: the
detector array would then be transported physically between the two positions,
depending on the resolution required.
Moderator.
Experience at existing pulsed neutron sources shows that a liquid methane-like
moderator is nearly ideal for this work, providing useful flux over a wavelength
range of at least 0.05Å to 4Å, while at the same time providing
an acceptable pulse shape. For liquid hydrogen the long wavelength spectrum
is enhanced, with poor pulse structure, making for substantial frame overlap
problems at 60Hz, while for a water moderator the flux cuts off at too
small a wavelength, around 2Å.
Choppers.
T0 and frame overlap choppers might be needed for this instrument, depending
on the moderator and flight path. Experience at existing pulsed sources
does not yet say whether a T0 chopper improves the background on a diffractometer
appreciably, and it has the disadvantage of limiting the minimum wavelength
to around 0.2Å. By the time L&AD begins detailed design, the
necessary tests will have been completed at ISIS. And additional information
will be forthcoming from experience gained from the HIPPO instrument under
construction at LANSCE.
Scattering
Angles. There should be maximum solid angle coverage at
all chosen angles to exploit the full capabilities of the SNS. The majority
of liquids diffraction is best performed at low angles in the range 0 -
60 in order to minimize inelasticity corrections. Special sample environments
and high resolution dictate that detectors at 90 and in backscattering
geometry should be provided. The 90 detectors will also allow measuring
Q perpendicular and parallel to aligned films. The backscattering detectors
should be full cone, highly pixelated for texture measurements. These requirements
mean that the instrument should be perpendicular to the moderator. (If
anomalous scattering is to be performed, then the angular range should
be continuous with no abrupt changes in resolution?)
Resolution.
The table below lists the required resolutions (Q/Q) for the two instrument
options.
2 |
Q-range |
Low resolution option |
High resolution option |
0 - 5 |
0 - 1Å-1 |
~5% |
~5% |
5 - 60 |
1 - 50Å-1 |
~2 - 3% |
2% |
90 |
2 - 100Å-1 |
0.7% |
0.4% |
150 |
3 - 100Å-1 |
0.5% |
0.25% |
The low resolution option would be realized
with a diffractometer at ~10 to 11 m from the target, while the high resolution
option would require a minimum of 17m, subject to detailed moderator specification.
These flight paths are dictated by the intrinsic pulse widths from the
source: narrowing the pulse (by means of thin moderators for example) too
much on a short flight path instrument to achieve better resolution throws
away the enhanced flux that would otherwise be achieved at SNS and is therefore
not acceptable.
Detectors and
monitors. Although detailed design cannot be performed at
this stage, several requirements are paramount to the success of the instrument
and will require detailed study well ahead of the instrument being designed
in detail. The detectors must be as efficient as possible, to exploit
the high neutron energies available at SNS. They must be fast to
accommodate the extremely high count rates (>1MHz) at short flight times.
They must be stable in order to be able to exploit the high flux
(0.1% drift in efficiency over 24 hours is a minimum requirement, but ideally
should be better than this). And they must be
insensitive to radiation.
In addition suitable beam monitors will be needed before and after the
sample position to monitor the flux incident on the sample, and the neutron
attenuation by the sample, respectively. Detector development needs to
begin very soon if SNS is to be viable by 2005.
Other aspects
and data analysis. Numerous other aspects of the diffractometer
will need to be specified, such as appropriate sample environment, beamline
collimation and shielding, access to the sample position and data acquisition
electronics. These are likely to be common to many SNS instruments, and
will need to be considered across the facility. A particular requirement
for this instrument is for a multiple position, temperature controlled,
sample changer for rapidly moving between samples, in situations where
the very highest resolution is required. A full range of standard equipment
e.g. cryostats, furnaces, pressure, magnetic/electric fields, gas flow
etc. Adjustable apertures should be provided on the incident beam, and
converging collimators should be investigated as an option. These would
be inserted when the very low Q-values are required. Equally important
will be the provision of an appropriate, comprehensive and user-friendly
data analysis package to assist users in interpreting their data.
Other instruments
(Back)
There are a number of other instruments which
are used by the disordered materials community with some frequency. Although
these instruments are being championed by other focus groups, there should
be input from the disordered materials community into the design of these
instruments for the SNS. Some of these beamlines will be baseline instruments
available on startup, others would be more appropriate for a second target
station (indicated by *).
Small angle
scattering.* The SANS
instrument should have a Q-range from 0.001Å-1 to 2Å-1.
There should be a decade in Q overlap between the 2 instruments with comparable
resolution in the Q range spanning the first peak in S(Q) (say, 2 Å-1).
Resolution in this region to be about 2%, which is essential for normalization
between the two instruments.
Anomalous scattering.
If
anomalous scattering is not possible on the L&A diffractometer, then
a chopper instrument with full angular coverage could be used in diffraction
mode.
Inelastic instruments.
The
main requirement is for a chopper instrument for S(Q,w) measurements with
an energy range up to several 100 meV with resolution better than 1%. It
should have a full range of continuous angles and a low angle detector
for Brillouin scattering.
Quasi-elastic
scattering.* There could
be many instruments in this category with energy resolutions ranging from
1µev to 50µeV each with a wide energy range. It might be best
to concentrate on one instrument in the first instance. Perhaps this should
be the one with 1µeV resolution. The instrument should have wide
angular (Q) coverage with a dedicated small angle arrangement.
Spin-echo instrument.*This
is also an attractive possibility for exploring such areas as the alpha
relaxation and nanosecond motions in proteins.
Polarization.The
community would like to be kept aware of developments in polarization analysis
instruments. These would be used for example for separation of incoherent
& coherent scattering.
Total scattering.
The
ability to use another instrument or beam line for transmission experiments
in order to measure total cross-section would be useful.
Elastic diffraction.Inelastic
instruments could also be used for measuring elastic diffraction by measuring
at zero energy transfer.
References
-
"ESS, A Next Generation Neutron Source for
Europe", Vol II. The Scientific Case. John L. Finney, co-ordinator, University
College London, March 1997.
Appendix
A. Current And Potential Research to Be Conducted at the SNS by the Liquids
and Disordered Materials Science Community (Back)
-
The Intermolecular Hydrogen-Hydrogen
Structure in Polymers from Neutron Diffraction (B. K. Annis, J.
D. Londono, , A. K. Soper)
-
Aqueous Solutions to High
Temperatures and Pressures (J. M. Simonson, ORNL)
-
Determination of Local Environment
of Lithium Ions in a Polymer Based Electrolyte (B. K. Annis, ORNL,
J. D. Londono, DuPont, J. Z. Turner, ISIS)
-
Boson peaks in disordered
materials (B. K. Annis, ORNL)
-
Conducting polymers electrolytes
(M-L. Saboungi, D. L. Price, ANL)
-
Non-aqueous electrolyte
solutions (W. S. Howells, RAL, ISIS)
-
QENS In Bulk Supercooled
and Interfacial Water (S.H. Chen, MIT)
-
Neutron Brillouin Spectroscopy
Of High-Frequency Collective Modes in Bulk and Interfacial Water
(S.H. Chen, MIT)
-
Dynamics of Bulk water under
extreme conditions of temperature and pressure (M-C. Bellissent-Funel,
Laboratoire Leon Brillouin)
-
Role of water in biological
macromolecules (M-C. Bellissent-Funel, Laboratoire Leon Brillouin)
-
Super-ionic glasses
(Lars Borjesson, Chalmers University of Technology)
-
Vibrational density of states
for network glasses (Adrian Wright, University of Reading)
-
Geophysical materials: silicate
liquids and glasses (M. Wilding, UC Davis)
-
Microscopic Structure of
4He-3He Mixtures (P.Sokol, Penn State U)
-
Collective Excitations in
Confined Superfluid Helium (P. Sokol, Penn State U)
-
PDF Analysis Applied to
Disordered Crystals (T. Egami, University of Pennsylvania)
-
Structured Ion Conductors
(J. W. Zwanziger, Dalhousie University)
-
Critical Micelle Conditions
in Supercritical CO2 (H. D. Cochran, G. D. Wignall,
ORNL, J. D. Londono, DuPont)
-
Chain Molecules in Supercritical
CO2 (H. D. Cochran, G. D. Wignall, Y. B. Melnichenko,
ORNL)
-
Chain Molecule Systems Under
Shear (H. D. Cochran, H. J. Dai, ORNL, M. D. Dadmun, U. Tenn.)
-
Structure Property Relations
in Transition Metal Oxides (S. J. L. Billinge)
-
Thermodynamics from diffraction
(S. J. L. Billinge, P. G. Radaelli)
-
Aqueous Electrolyte Solutions:
Structural Studies (G. W. Neilson, Bristol)
-
Local Structure Studies
of Pi-conjugated and Other Unconventional Polymers (M. J. Winokur,
University of Wisconsin-Madison; B.R. Mattes, Los Alamos National Laboratory)
-
Glass Transitions
(F. J. Bermejo, CSIC- Madrid) )
-
The Intermolecular Hydrogen-Hydrogen Structure in Polymers from Neutron
Diffraction (B. K. Annis, J. D. Londono, , A. K. Soper) -- Interchain
interactions in amorphous polymers influence static quantities such as
the cohesive energy density and miscibility as well as dynamic processes
such as chain mobility and possibly the behavior of boson peaks. Neutron
scattering experiments using H-D isotopic substitution permit the direct
determination of the intermolecular H-H structure function hHH(Q)
and the intermolecular H-H correlation function gHHinter(r)
without recourse to models of the
intramolecular structure. The
method is unique for obtaining this kind of information in polymers and
permits determination of the global "correlation hole" where gHHinter(r)
< 1 for a considerable range, due to the screening of intermolecular
correlations by intramolecular correlations. By suitable H-D substitution
on side groups, the method can in principle probe the reduction in interchain
correlation specific to these groups, i. e. the "local correlation hole".
The resulting distribution functions provide stringent tests of molecular
dynamics simulations and theoretical models. These studies require high
intensity to obtain sufficient statistics in the difference function and
very good detector stability. They also have detector placement requirements
not available on typical powder diffraction instruments and beamlines optimized
for scattering from hydrogenous liquid/amorphous materials. These needs
argue for a liquid/amorphous diffractometer at the SNS.
-
Aqueous Solutions to High Temperatures and Pressures (J. M. Simonson,
ORNL) - The large qualitative changes in the behavior of aqueous solutions
with increasing temperature are driven by changes in the local structure
near solute particles (e.g., hydration or ion pairing) coupled with the
strong increase in the compressibility of the solvent. Quantitative, predictive
understanding of these phenomena, needed to model the behavior of these
fluids in natural and technological environments, requires that these local
structural and dynamic changes be measured precisely over wide ranges of
state conditions (temperature, pressure, and composition). While the method
of neutron diffraction with isotopic substitution has been shown to give
the information needed under favorable conditions, progress is hindered
by limitations of current facilities for the study of inherently low-contrast,
high-background experiments such as dilute solutions in heavy-walled pressure
vessels at high temperatures. New advances in experimental information
available through the high flux of the SNS, coupled with a highly-stable,
moderate-resolution diffractometer optimized for the study of short-range
order in noncrystalline materials, will enable studies of additional elements
having too little contrast for investigation on present facilities and
will give the levels of precision and accuracy needed to develop and refine
molecular-scale models and simulations of the properties of solutions under
extreme conditions.
-
Determination of Local Environment of Lithium Ions in a Polymer Based
Electrolyte (B. K. Annis, ORNL, J. D. Londono, DuPont, ) -- Electrolytes,
composed of lithium salts dissolved in a polymer, are currently considered
to be candidates for battery development . The cation conduction mechanism
in these rubbery materials is not well understood, and will depend on the
local environment of the ion. The radial distribution function about Li+
has been determined by the single difference method, using two solutions
of LiI in deuterated polyethylene oxide (PEO). The solutions were measured
at a temperature of about 90oC at which point the substantial
association of ether oxygens to Li+ is expected. The number
of associated oxygen atoms was found to be similar to that in the crystalline
phase. Studies of this type need to be carried out at a variety of temperatures
and lithium ion concentrations and would benefit greatly from the high
throughput available on a high intensity diffractometer. The effect of
the ions on the polymer chain configuration can be determined by accurate
measurements of radius of gyration which would be a SANS problem.
-
Boson peaks in disordered materials (B. K. Annis, ORNL) -- A peak
in scattered intensity in the range 1-5 meV in the vicinity of the glass
transition and below it is known as the boson peak. The understanding of
the origins of this peak is a matter of much exsperimental and theoretical
investigation. Although it is a nearly ubiquitous phenomenon in disordered
materials, the intensity, temperature dependence and position of the peak
varies significantly with the specific material. Study of the boson peak
requires spectra collected at several temperatures. In the case of the
so-called "fragile" glass formers it is expected that pressure mapping
of the peak position will also be of interest. The instrumental requirements
are an energy resolution of 50 microeV, and energy range out to at least
10 meV and a Q range of 0.5-3.0 -1.
-
Conducting polymers electrolytes (M-L.
Saboungi, D. L. Price, ANL) -- Among ionic conducting materials, PEO mixed
with various salts, is considered to be a typical solid polymer electrolyte
with a number of potential technological applications such as advanced
batteries, sensors and electrochromics. PEO can form complexes with various
salts including those of alkali metals and alkaline earths. However their
development has been somewhat hindered by the limited knowledge about their
properties, especially the nature of their ionic conduction in terms of
microscopic structure and dynamical behaviour. Neutron diffraction (with
isotopic substitution) can elucidate the structure and QENS will then probe
the dynamics, in particular the local chain motion and how it is affected
by the ions.
-
Non-aqueous electrolyte solutions (W.
S. Howells, RAL, ISIS) - Non-aqueous solutions have applications in batteries
, electro-deposition of metals, production of wet capacitors and in electro-organic
synthesis. The solvents (such as dimethyl sulphoxide, acetonitrile and
methanol) are important industrial solvents as well as being widely used
in organic chemistry, fine chemical technology and biology (DMSO is a cryoprotectant
for biological structures). These solutions are also a severe test for
current theories due to the absence of quantitative information about the
specific ion-molecular interactions and the influence of the ion on molecular
dynamics, structure and strength of intermolecular interactions in solutions.
Neutron diffraction with isotopic substitution will determine the structure
and QENS will probe the dynamics of the protons in the hydrogenous solvent
and investigate the changes with, for example, type of ion and concentration.
The results can then be compared directly with molecular dynamics simulations.
-
QENS In Bulk Supercooled and Interfacial
Water (S.H. Chen, MIT) - Presence of "interfacial water" in proteins,
DNAs and membranes plays a fundamental role in triggering the "slow dynamics"
in these bio-macromolecules which makes various enzymatic and biological
functions possible. There is a strong evidence that dynamics of the interfacial
water is similar to a bulk supercooled water at a lower temperature. In
bulk supercooled and interfacial water, the test-particle intermediate
scattering function shows a two-step (fast and slow) relaxation with a
plateau in-between. This plateau defines an incoherent Debye-Waller factor
which is a measure of the size of the transient "cage" surrounding the
water molecule. The fast relaxation decays in less than a ps and can be
attributed to rattling motions of water molecules in the cage. These vibrations
are harmonic with a density of states which has a two-peak structure. The
slow relaxation is approximately stretched exponential having a relaxation
time in the hundreds of ps or in ns (slow dynamics). High resolution QENS
spectra are dominated by the slow relaxation which reflects largely the
CM motions of water molecules following the structural re-arrangement of
the cage. The resulting QENS line shape is non-Lorenzian. The QENS spectrometer
should be designed with an optimal energy resolution in the several µV
range in order to study the detail of the line shape. It should have a
Q coverage of Qmin = 0.1 Å-1 and Qmax
= 2.5 Å-1 in order to reach the diffusion limit at the
lower end and to detect the Q-dependent shape of the D-W factor at the
higher end.
-
Neutron Brillouin Spectroscopy Of High-Frequency
Collective Modes in Bulk And Interfacial Water (S.H. Chen, MIT) - Water
shows an anomalous dispersion of sound waves at a q of about 0.3 Å-1,
above which the sound speed increases by a factor two. In order to study
the excitation, incident neutron energy has to be greater than 80 meV.
The excitation persists up to at least q = 1.0 Å-1. INS
spectrometer needs to have 0.5% energy resolution at 100 meV and a Q coverage
of 0.1 -1 to 2.0 Å-1, in order to be competitive
with IXS. The coherent Rayleigh-Brillouin triplet at the above mentioned
q range consists of a strong central peak and a weak side wings, which
are due to the existence of the high-frequency sound. In order to study
the spectral width and shape of the central peak, one needs to use a spin
echo type spectrometer with a time range of 1 ps to 20 ns. This is because
the alpha-relaxation process, which gives rise to the central peak, has
a relaxation time which show a strong q-dependence.
-
Dynamics of Bulk water under extreme conditions
of temperature and pressure (M-C. Bellissent-Funel, Laboratoire Leon
Brillouin) - This research activity is in relation with the structural
studies of water that has been investigated in a wide range of temperatures
and pressures. Some information about the degree of hydrogen bonding is
now available: in supercritical states -- M-C. Bellissent-Funel et al.,
JCP, 107, 2942 (1997), T. Tassaing et al., Europhys. Let., 42, 265 (1998);
in supercooled states : M.-C. Bellissent-Funel et al., JCP, 102, 3727(1995),
M.-C. Bellissent-Funel, Europhys. Let, 42, 161 (1998). There is a big interest
to study dynamics of water by quasielastic neutron scattering for the same
pressures and temperatures. The whole structural and dynamics information
about water as functions of temperature and pressure could allow to access
to the more reliable potential among the various water potentials that
are presently available.
-
Role of water in biological macromolecules
(M-C. Bellissent-Funel, Laboratoire Leon Brillouin) - An important field
of research in protein biophysics concerns the role of water on the structure,
dynamics and function of globular protein. Both the effect of water on
internal protein motions and the effect of protein on surrounding water
dynamics are of primary concern in these studies.
-
Dynamics of Water at Model Interfaces -
hydrophilic surfaces such as silica surface (porous Vycor glass). From
structural and dynamic studies by neutron scattering, there is a strong
evidence that surface water behaves like a bulk supercooled water, M.-C.Bellissent-Funel
et al, JCP, 98, 4246 (1993) (Structure), M.-C.Bellissent-Funel et al, PRE,
51, 4558 (1995). The single particle motions are now analyzed in terms
of the alpha relaxation dynamics predicted by mode coupling theory of supercooled
liquids, J.-M. Zanotti et al, PRE (1998).
-
Dynamics of Water at surface of a protein.
Some
similar results are obtained at the surface of a deuterated C-phycocyanin
protein., M.-C.Bellissent-Funel et al, Faraday Discuss.,103, 281, (1996),
S. Dellerue et al, to be published.
-
Dynamics of proteins at different time
scales. Quasielastic and inelastic neutron scattering allows the investigation
of motions ranging from subpicoseconds to nanoseconds. That can be achieved
by changing the resolution of the instruments. Vibrational motions, picosecond
diffusion motions of surface residues have been observed in soluble globular
proteins such as C-phycocyanin, parvalbumin, lyzozyme as well as in membrane
proteins. Nanosecond motions in the same proteins are under investigation
by neutron spin-echo technique (Thesis of S.Dellerue in preparation).
-
Super-ionic glasses (Lars Borjesson,
Chalmers University of Technology) - Super-ionic glasses are solid materials
where some ionic species are mobile within an otherwise frozen glassy network.
These materials are thus of highest interest for electrochemical applications
as solid electrolytes e.g. in solid-state batteries, sensors or smart-windows.
These glasses also represent a challenge from a more fundamental point
of view. The mechanism behind the high conductivity in this material is
far from trivial and despite extensive research for the last decades there
is no macroscopic theory explaining the conduction process. the development
of new materials is so far based on experience and common practice rather
than on fundamental understanding of the materials. Neutron diffraction
has already established that these glasses have an expanded structure leading
to conduction pathways. QENS is then able to investigate the mobility of
the conducting ions.
-
Vibrational density of states for network
glasses (Adrian Wright, University of Reading) - An investigation of
the sharp features in the vibrational density of states for network glasses
arising from superstructural units and other small planar rings, including
the Q-dependence (as an aid to their interpretation). N.B. A quantitative
study cannot be performed by Raman Spectroscopy, due to unknown and large
matrix element effects. From the point of view of inorganic network glasses,
the greatest step forward in inelastic scattering instrumentation at ISIS
has been the MARI spectrometer since, for the first time, this has the
resolution necessary to investigate the sharp peaks arising from superstructural
units (e.g. boroxol and triborate groups) in borate glasses and the sharp
lines in other systems resulting from small planar rings, of the type investigated
by Frank Galeener using Raman scattering. It also has a wide angle coverage,
which makes it possible to investigate the Q-dependence of these modes
- at least it would if sufficient time were available on the instrument!
Similarly, the fact that the time per sample on MARI is 4-6 days means
that it is not feasible to carry out extensive studies as a function of
composition, which is extremely important in the case of binary and multicomponent
glasses. These experiments will become feasible at the SNS.
-
Geophysical materials: silicate liquids
and glasses (M. Wilding, UC Davis) - Silicate liquid behavior influences
a variety of geochemical and geophysical processes. Although such liquids
have a complex evolution over a range of pressure temperature and composition,
considerable insight into their behavior can be obtained through study
of simple silicate liquids and glasses that contain elements of geochemical
interest. Of particular importance are silicate systems, which contain
rare earth and transition metal elements. Thermodynamic measurements can
be used to establish the macroscopic influence of such components but their
mechanism of dissolution remains uncertain. Previous neutron scattering
studies have provided important information on the structural role of Ca,
Ti, Ni and Li in silicate glasses. These studies have used elements with
isotopes of sufficiently different scattering lengths to enable study by
isotopic substitution. The advent of the SNS with increased intensity,
increased range of Q, increased resolution and potential for additional
compositions available for isotopic substitution, provides an opportunity
to expand this data base. The focus of such new studies would be to evaluate
the structure of multicomponent glasses as a function of composition; to
carry out phase diagram-based studies and to study the differences between
liquid and glassy states. If combined with complementary spectroscopic
techniques such as NMR, neutron scattering studies can provide a rigorous
structural base for independent thermodynamic probes of such molecular
liquids. Such developments will contribute to the understanding of a variety
of processes of interest to the Earth Science Community, including the
temperature-dependent rearrangement of melt structure, the solubility of
H2O in silicate liquids, crystallization and melting processes,
reactions at mineral surface, pre-melting of minerals and hydrothermal
alteration.
-
Microscopic Structure of 4He-3He Mixtures
(P.Sokol, Penn State U) - The microscopic properties of pure 3He-4He mixtures
are of considerable interest. Understanding these microscopic properties,
such as the Bose condensate fraction, present a considerable challenge
since they now involve quantum exchange effects among particles obeying
different statistics. This statistical disorder leads to a variety of new
behaviors, such as depression of the superfluid transition and suppression
of the superfluid fraction. Recent experimental measurements using high
energy inelastic neutron scattering have yielded surprising results, such
as an increase of the Bose condensate fraction no, which is the microscopic
order parameter for the superfluid phase, on the addition of 3He. Measurements
of the kinetic energy per atom (KE) have suggested that the local environment
of the atoms in the mixture is quite different from what any of the current
theories predicts. Neutron structure measurements, in combination with
x-ray measurements, can provide direct information on the various pair
distribution functions for the mixture and elucidate the local structure
of the liquid. High intensity, due to the high absorption cross section
of 3He, and excellent statistical accuracy, due to the small differences
in the local environment of the isotopes, will be required.
-
Collective Excitations in Confined Superfluid
Helium (P. Sokol, Penn State U) - Confinement of helium in disordered
environments introduces profound changes in the behavior of the superfluid
phase. When helium is liquefied in Vycor glass (with highly interconnected
pores of less than 100 Å diameter), for example, the lambda transition
is suppressed by about 0.2K, but the superfluid density exponent n is essentially
unchanged from its bulk value (~0.67). For aerogel glass, which has a very
open structure with a wide range of pore diameters, Tl is only suppressed
by <0.01K whereas n is markedly increased (to ~0.81) indicating that
the disordered helium now belongs in a different universality class. The
disorder introduced by confinement affects many other macroscopic properties
such as heat capacity, vorticity, and flow. An understanding of the microscopic
dynamics, obtained through inelastic neutron scattering, is fundamental
to developing a complete picture of these unique liquids. One of the most
direct ways these microscopic dynamics are manifested is in the collective
excitations, which are the fundamental excitations of the system and are
directly related to the macroscopic properties of the liquid, such as the
heat capacity and superfluid fraction. In superfluid helium, for example,
these collective excitations are the well-known phonon-roton dispersion
relation. The nature and interactions of these elementary excitations play
a major role in determining the macroscopic properties of both the normal
and superfluid phases. Measurements of the differences introduced by confinement
will require excellent energy (~5-10 ueV) and momentum (~ 0.01-0.05 A-1)
resolution.
-
PDF Analysis Applied to Disordered Crystals
(T. Egami, University of Pennsylvania) -- Many of today's advanced materials
have complex structures with some degree of disorder at the atomic as well
as the mesoscopic scale. Examples include the magnetic oxides with giant
magnetoresistance, superconducting cuprates, ferroelectric and relaxor
ferroelectric oxides, catalytic and catalytic support oxides, semicrystalline
polymers, nanocrystalline particles, and semiconductor alloys. The traditional
crystallographic approach has a very limited power in determining the structure
of these materials. A powerful alternative is to apply the atomic pair-density
function (PDF) analysis. Indeed our studies on many of the materials listed
above resulted in many discoveries and novel understanding of the structure-properties
relation. The pulsed neutron diffraction has been the best method to obtain
the PDF, since the epithermal portion of the neutron spectrum provides
ample neutrons with short wavelengths. By using the pulsed neutron sources
we can determine the structure function up to 40 - 50 A-1, eliminating
much of the termination errors from the Fourier-transform to obtain the
PDF. The requirement of the instrument for such a purpose is the high intensity
and the high resolution (< 0.2 %). Without high resolution the features
at high Q will be lost, resulting in the loss of real space resolution
in the PDF. The accuracy of the PDF analysis has been improved to the extent
that it is equivalent in accuracy to the Rietveld powder diffraction analysis
in determining the atomic positions in complex crystals. The new science
that can be conducted at the SNS includes:
-
The study of the local and mesoscopic structure
of CMR oxides. We now understand the local structure of the CMR oxides,
but how the local modifications (polarons) organize themselves in the meso-scopic
scale is still open. Higher intensity and higher resolution will be needed
to advance the PDF to the next stage to allow the meso-scopic structure
study.
-
Local structure of electronic ceramics, including
the oxide with metal- insulator transition. The mechanism of the metal-insulator
transition appears to involve the local lattice modifications in many cases.
Determination of the local as well as mesoscopic structure through the
metal-insulator transition will greatly advance our understanding.
-
Local structure of nano-crystalline ceramics
produced by bio-directed sol-gel processing. Here the mesoscopic nucleation
of crystalline phases in the gel is the key in this process. Since the
nuclei are small (although high in density in this method), conventional
method will not be able to determine their structure.
-
Local and meso-scopic structure of biological
systems including proteins. The crystallization process used in protein
crystallography often modifies the protein folding and its functionality.
The PDF method allows the direct determination of the real space distances
in the liquid solution so that the real structure of proteins can be understood.
-
Structured Ion Conductors (J. W. Zwanziger,
Indiana University) - The ability to structure materials on the nanoscopic
scale through self-assembly techniques is opening new opportunities in
materials science. One exciting approach is to use block-co-polymers as
the structure directing agent, taking advantage of the mesophase separation
into ordered arrays that is the thermodynamic equilibrium of these materials.
We have developed a system for lithium ion conductivity, in which polyethylene
oxide is used as one arm in a block-co-polymer. The PEO thus can be used
to do double duty as both structure director and pathway for conduction.
Our goal with this material is to use it as a solid electrolyte in batteries,
in particular micro-batteries which could employ self-assembled ion-conducting
lithium wires. To develop and exploit this system we need to understand
both the structure and dynamics in the material, and neutron diffraction
will be an essential part of his goal. Specifically, because of the multiple
length scales and disorder within the polymer blocks, we require instrumentation
suitable for disordered material diffraction which also has a significant
overlap at low scattering vector with traditional small angle neutron diffractometers.
At the SNS we have the opportunity to build such an instrument, which would
be unique in the field and would make the SNS the premier facility world-wide
for investigation with neutrons of mesocopically structured materials.
-
Critical Micelle Conditions in Supercritical
CO2 (H. D. Cochran,
G. D. Wignall, ORNL, J. D. Londono, DuPont) -- We currently study the formation
of micelles in supercritical CO2 using the SANS instrument at
HFIR. These fundamental studies have great potential for practical application
because the chemical industry is actively seeking ways to use supercritical
CO2 to replace organic solvents, and already a number of processes
for polymerization within micelles in CO2 are under development.
It has been found that in supercritical CO2 there is a critical
micelle density (analogous and in addition to the familiar critical micelle
concentration in incompressible liquid media) below which micelles form
and above which surfactant molecules are dispersed in solution. However,
the surfactant concentrations and nascent micelle sizes of interest in
studying the self-assembly of micelles demand wide angle neutron scattering
experiments to complement SANS and higher flux than HFIR can provide. This
is one of several efforts aimed at bridging the gulf between understanding
of complex fluids at the atomic scale and their important properties at
mesoscale through coupled scattering and molecular simulation studies.
-
Chain Molecules in Supercritical CO2
(H. D. Cochran, G. D. Wignall, Y. B. Melnichenko, ORNL) - With SANS experiments
we have studied variation of the radius of gyration of polymer chains in
supercritical CO2. These experiments allow us to study the theta
condition of polymer solutions as a function of density as well as temperature
(usually the density effect is small in incompressible liquids). Both molecular
simulations and statistical mechanically-based equations of state for polymer
solutions can be most effectively tested with systems in which both density
and temperature can be widely varied. The systems amenable so far to experimental
study have been too large and complex for complementary atomistic molecular
simulations. Thus, there is an incentive to pursue complementary wide angle
neutron scattering experiments with simpler, smaller molecular structures
(requiring lower concentrations and maximum neutron flux). This is one
of several efforts aimed at bridging the gulf between understanding of
complex fluids at the atomic scale and their important properties at mesoscale
through coupled scattering and simulation studies.
-
Chain Molecule Systems Under Shear
(H. D. Cochran, H. J. Dai, ORNL, M. D. Dadmun, U. Tenn.) - We are building
an ultra-high strain rate shear cell for scattering studies of the structure
of chain molecule systems. The aim is to achieve overlap of strain rate
between experiments and accurate, atomistic non-equilibrium molecular dynamics
calculations. Currently, the highest strain rate achieved in carefully
controlled experiments is <107s-1, and the lowest
strain rate achieved in an accurate, atomistic simulation is >108s-1.
Before simulation can reliably be used to test new molecular- level theories
of fluids under shear flow, the quantitative accuracy of the simulations
must be verified for real fluids. The first experiments will employ laser
light scattering, but ultimately x-ray scattering and (hopefully) neutron
scattering may be attempted. The requirement of extremely high strain rate
in shear implies extremely short path length through the sheared sample,
which will require the highest available neutron flux. Based on laser and
x-ray results, the feasibility of neutron experiments at the SNS will be
evaluated. This is one of several efforts aimed at bridging the gulf between
understanding of complex fluids at the atomic scale and their important
properties at mesoscale through coupled scattering and simulation studies.
-
Structure Property Relations in Transition
Metal Oxides (S. J. L. Billinge) -- Transition metal oxides have a
wealth of interesting and potentially useful properties such as high- temperature
superconductivity, ferroelectricity and colossal magnetoresistance. These
come about because of interesting interplays between electronic and structural
effects. Often structural distortions which strongly affect the properties
are present which are disordered and cannot be studied crystallographically.
We will be advancing high- resolution real-space techniques such as the
atomic pair distribution function (PDF), and single crystal diffuse scattering
techniques at the SNS to study these effects. High Q- resolution and a
wide Q-range are necessary for these measurements as well as low backgrounds
and stable moderator/detector systems and excellent statistics. This requires
a spallation source with high flux and a dedicated instruments designed
with these parameters in mind.
-
Thermodynamics from diffraction (S.
J. L. Billinge, P. G. Radaelli) -- A great deal of information about a
material can be obtained from a small number of measurements and the application
of the principles of thermodynamics. For example, the measurement of specific
heat gives important (though incomplete) information about the density
of states of a sample and therefore the bonding. This information is also
stored in the thermal vibrational amplitudes of solids which can be measured
using diffraction. We are reaching the point with instrumentation and analysis
techniques that absolute displacement factors can be determined, as well
as their temperature dependence, from powder diffraction. This can be done
using Rietveld refinement, or refinement of the real- space pair distribution
function. Rietveld is straightforward and yields the uncorrelated thermal
factor which is a weighted sum over all the modes in the material. The
PDF yields bond-specific thermal vibrations which give information about
specific optical vibration modes. These values can be analyzed to obtain
information about the bonding in materials without having to carry out
difficult and time-consuming phonon dispersion measurements on single crystals,
for example. For accurate absolute thermal factors, proper corrections
for effects such as absorption and multiple scattering have to be made
making an instrument with stable characteristics and low backgrounds important.
-
Aqueous Electrolyte Solutions: Structural
Studies (G. W. Neilson, Bristol) - Aqueous electrolyte solutions exhibit
a rich variety of properties over a wide range of thermodynamic phase,
and are significant components in many geological and biochemical fluid
systems. Neutron diffraction when combined with isotopic substitution (NDIS)
provides the only means currently available to determine the detailed interatomic
structure around ions and water molecules in solution. A suitably configured
and optimized diffractometer on the SNS will enable more extensive investigations
to be carried out at lower ionic concentrations and into regimes of more
biological significance. It will also allow for the determination of the
structure around ions of elements with differences in neutron scattering
lengths which are relatively small and not yet susceptible to the NDIS
method (e.g. ions of Sn, U, Hf, Pb, etc). In the longer term, experiments
may be successfully undertaken to monitor relatively slow but well-defined
kinetic processes, or in the study of ionic coordination in the neighborhood
of an electrode/electrolyte interface.
-
Local Structure Studies of Pi-conjugated
and Other Unconventional Polymers (M. J. Winokur, University of Wisconsin-Madison;
B.R. Mattes, Los Alamos National Laboratory) - Conducting, pi-conjugated
polymers continue to undergo a remarkable evolution in terms of their synthesis,
processing, and materials properties. As a direct result of this focused
effort there are now a wealth of new applications (highly selective separation
membranes, biosensors, corrosion resistant coatings, light emitting diodes,
microacutators) in addition to the many ``conventional'' uses originally
envisioned. Despite the emphasis that is often placed on the quasi-one-dimensional
nature of the host materials and the accompanying electronic excitations,
it is the microscopic molecular organization and the precise control of
this structure, with respect to both the intra-chain and inter-chain order,
that often defines and ultimately limits these materials. Even relatively
small changes in the specific chemical architecture and/or processing can
lead to significant variations in the resultant structural forms and in
their physical properties. With the fabrication of increasingly more complex
architectures, there is an acute need to identify what are the local structural
characteristics and which attributes play a key role in influencing the
various structure/property relationships. One particularly notable family
of materials is polyaniline and its related derivatives. Polyaniline films
can be prepared as either amorphous or semicrystalline materials simply
by changing the extent of hydrogen boding inhibitor when dispersed in a
solution. Nominally identical crystalline materials can be prepared which
range from insulating to metallic. Resolving systematic variations in the
local intra-chain structure and intermolecular packing through pair-distribution
analysis of the structure function is essential if this diverse structural
behavior is to be fully understood. At present there is no US based neutron
facility available for effectively undertaking many these studies. Some
preliminary work has been begun using the SANDALS spectrometer (at ISIS)
but the improved capabilities presented by the SNS, through construction
of the liquid and disordered solids spectrometer, would dramatically extend
the range of studies that could be accomplished.
-
Glass Transitions (F. J. Bermejo, CSIC-
Madrid) -- Although extensively studied during the last decades, the dynamics
of the liquid -> glass transition remains as a poorly understood and a
highly controversial. Part of the difficulties are of instrumental nature:
the need to perform measurements over frequencies comprising many decades
and the unsurmountable problem of combining data measured using wildly
different time-windows. Future neutron sources can surely contribute towards
the advance of our knowledge by : a) the development of backscattering
(BS) spectrometers with nano-eV resolution, achievable using Bragg angles
close to 90 deg and crystals such as GaAs; b) the development of BS spectrometers
with active monochromators which will improve the appalling Q-resolution
of the present-day spectrometers and c) the development of offset monochromators
(J.C. Cook et al. Nucl.Inst. Meth. A312, 553 ,1992) which will enable the
exploration of energy transfers up to at least 1 meV still retaining micro-eV
resolution.
Appendix B. Organizing Committee
(Back)
Price, David L., Argonne National Laboratory, 9700 S.Cass Avenue, Argonne
IL 60439,
price@anlpns.pns.anl.gov,
phone: 630-252-5475, fax: 630-252-7777
Annis, Brian K., Oak Ridge National Laboratory, P. 0. Box 2008, Oak
Ridge TN 37831-6197,
annisbk@ornl.gov,
phone: 423-574-5047, fax: 423-576-5235
Saboungi, Marie-Louise, Argonne National Laboratory, 9700 S. Cass Avenue,
Argonne lL 60439, saboungi@anlpns.pns.anl.gov,
phone: 630-252-4341, fax: 630-252-7777
Habenschuss, Tony, Oak Ridge National Laboratory, P. 0. Box 2008, Oak
Ridge TN 37831-6197, tth@ornl.gov, phone.
423-574-6018, fax: 423-576-5235
Appendix C. Workshop Agenda
(Back)
SNS Workshop on Disordered Materials, 16-17 Oct 1998
Sponsored by Argonne and Oak Ridge National Laboratories
Building 360, Argonne National Laboratory, 9700 S. Cass Avenue, Argonne,
IL 60439
Friday, October 16, 1998
8:30 Coffee and Continental breakfast
9:00 WORKSHOP GOALS Bruce Brown (Director, IPNS)
Orientation: REVIEW OF SNS DESIGN Chair: Bruce Brown
9:10 Source, target(s), moderators Jack Carpenter (ANL)
9:40 Instrumentation and data acquisition Kent Crawford (ANL)
10:10 Coffee Break
Session A: LIQUID STRUCTURE: PERFORMANCE REQUIREMENTS Chair: Brian Annis
(ORNL)
10:30 Hydrogenous liquids Marie-Claire Bellissent (Saclay)
10:50 High-temperature liquids Marie-Louise Saboungi (ANL)
11:10 Aqueous solutions George Neilson (Bristol University)
11:30 Liquid polymers Brian Annis (ORNL)
11:50 Review of HIPPO (diffractometer proposed for LANSCE) Bob
Von Dreele (LANL)
12:10 Lunch
1:10 Discussion of performance requirements for liquid structure Moderator:
Alan Soper (ISIS)
Session B: GLASS & DISORDERED SOLID STRUCTURE: PERFORMANCE REQUIREMENTS
Chair: Takeshi Egami (Univ. of Penn.)
2:10 Intermediate- and short-range order in glasses Adrian Wright
(Reading University)
2:30 Ternary glasses Joe Zwanziger (Dalhousie University)
2:50 Fast-ion conducting glasses Lars Borjesson (Chalmers Institute
of Technology, Goteborg)
3:10 Coffee Break
3:30 Geophysical materials Martin Wilding (UC, Davis)
3:50 Local correlations in solids Takeshi Egami (Univ. of Penn.)
4:10 Disordered polymers Michael Winokur (Univ. of Wisc.)
4:30 Small-angle scattering from porous materials Sow-Hsin Chen
(MIT)
6:00 Workshop dinner (Argonne Guest House)
Saturday, October 17, 1998
9:00 Coffee and Continental breakfast
9:30 Discussion of performance requirements for glass & disordered
solid structure Moderator: Spencer Howells (ISIS)
Session C: DYNAMICS OF DISORDERED MATERIALS: PERFORMANCE REQUIREMENTS
Chair: David Price (ANL)
10:30 Coffee Break
10:50 Soft matter Sow-Hsin Chen (MIT)
11:10 Quantum systems Paul Sokol (Penn. St. Univ.)
11:30 Vibrational spectroscopy Chun Loong (ANL)
11:50 Relaxation processes Franz Trouw (ANL)
12:10 Lunch
1:00 Discussion of performance requirements for dynamics of disordered
materials Moderator: Sow-Hsin Chen (MIT)
2:00 Breakout groups A, B, C (corresponding to sessions above) to draft
reports
4:00 Plenary meeting to discuss preparation of report and presentation
at SNS Users Meeting, Knoxville,
November 9-11, 1998. Chair: Brian Annis
5:00 Adjourn
Appendix D. List of Workshop
Participants (Back)
Annis, Brian, Oak Ridge National Laboratory, P. 0. Box 2008, Oak Ridge
TN 37831-6197, annisbk@ornl.gov phone:
423-574-5047, fax: 423-576-5235
Bellissent, Marie-Claire, Laboratoire Leon Brillouin, 3 Rue Michel Ange,
75974 Paris, France, mcbel@LLB.saclay.cea.fr
phone: 33+1-69-08-60-66, fax: 33+1-69-33--14-87
Borjesson, Lars, Chalmers University of Technology, S-412 96 Gothenburg,
Sweden, borje@fy.chalmers.se
phone: +46-31-772-33-07, fax: +46-31-772-20-90
Brown, Bruce, Argonne National Laboratory , 9700 S. Cass Avenue , Argonne
IL 60439, bsbrown@anl.gov phone: 630-252-4999,
fax: 630-252-4163
Carpenter, Jack, Argonne National Laboratory, 9700 S. Cass Avenue, Argonne
lL 60439, jmcarpenter@anl.gov
phone: 630-252-5519, fax: 630-252-4163
Chen, Sow-Hsin, Massachusetts Institute of Technology, 24-209, Dept.
of Nuclear Eng., Cambridge MA 02139, sowhsin@mit.edu
phone- 617-253-3810, fax: 617-258-8863
Cochran, Hank D., Oak Ridge National Laboratory, P. 0. Box 2009, Oak
Ridge TN 37831-6224, hdc@ornl.gov phone:
423-574-6821, fax: 423-241-4829
Crawford, Kent, Argonne National Laboratory, 9700 S. Cass Avenue, Argonne
IL 60439, rkcrawford@anl.gov phone:
630-252-7769, fax: 630-252-4163
Egami, Takeshi, University of Pennsylvania, 3231 Walnut Street, Philadelphia
PA 19104, egami@seas.upenn.edu
phone: 215-898-5138, fax: 215-573-2128
Habenschuss, Tony, Oak Ridge National Laboratory, P. 0. Box 2008, Oak
Ridge TN 37831-6197, tth@ornl.gov phone.
423-574-6018, fax: 423-576-5235
Howells, William Spencer, ISIS Facility. Rutherford Appleton Lab., Chilton,
Didcot, OX11 OQX, UK, wsh@isise.rl.ac.uk
phone: +44-1235-44-5680, fax- +44-1235-44-5720
Londono, David, E.I. DuPont de Nemours, P.O. Box 80323 Bldg. B323, Wilmington
DE 19880-0323, j-david.londono@usa.dupont.com
phone: 302-695-1222, fax: 302-695-1513
Loong, Chun, Argonne National Laboratory, 9700 S. Cass Avenue, Argonne
IL 69439, phone: 630-252-5596, fax: 630-252-4163
Neilson, George, H. H. Wills Physics Laboratory, Tyndall Avenue, Bristol,
BS8 1TL, UK, george.w.neilson@bristol.ac.uk
phone: 44-117-9288706, fax: 44-117-9255624
Price, David L., Argonne National Laboratory, 9700 S.Cass Avenue, Argonne
IL 60439, dlprice@anl.gov phone: 630-252-5475,
fax: 630-252-7777
Robertson, Lee, Oak Ridge National Laboratory, Bldg. 7962, MS 6393,
Oak Ridge TN 37831-6393, robertsonjl@ornl.gov
phone: 423-574-5243, fax: 423-574-6268
Saboungi, Marie-Louise, Argonne National Laboratory, 9700 S. Cass Avenue,
Argonne lL 60439, saboungi@anlpns.pns.anl.gov
phone: 630-252-4341, fax: 630-252-7777
Simonson, J. Mike, Oak Ridge National Laboratory, P. O. Box 2008, Bldg.
4500S, Oak Ridge TN 37831-6110, simonsonJM@ornl.gov
phone: 423-574-4962, fax: 423-574 4961
Sokol, Paul, Pennsylvania State University,, Dept. of Phys. 104 Davey
Lab., University Park PA 16802, pes4@psu.edu
phone: 814-863-5811, fax: 814-865-3604
Soper, Alan, ISIS, Rutherford Appleton Laboratory, Chilton, Didcot OXON
, OX11 OQX, UK, aks@isise.rl.ac.uk
phone: +44-1235-445543, fax: +44-1235-445642
Trouw, Frans, Los Alamos National Laboratory, Los Alamos NM, trouw@lanl.gov phone: 630-252-7665,
fax: 630-252-4163
Von Dreele, Robert, Argonne National Laboratory, 9700 S. Cass Avenue, Argonne, IL 60439vondreele@anl.gov
phone:, fax:
Wilding, Martin, University of California/Davis, Davis CA 95616, mcwilding@ucdavis.edu
phone: 530-754-2133, fax: 530-752-9307
Winokur, Michael, University of Wisconsin, Dept. of Physics, Madison
WI 53706, mwinokur@wisc.edu phone:
608-262-7475, fax: 609-265-2334
Wright, Adrian, J. J. Thompson Physical Laboratory, University of Reading,
Whiteknights Reading, RG6 6AF UK,
A.C.Wright@reading.ac.uk
phone: +44-119-931-8555, fhx: +44-118-975-0203
Zwanziger, Josef,Dalhousie University, Halifax, NS, B3H 4J3 CANADA
jzwanzig@dal.ca phone: (902) 494-1960,
fax: 902-494-1867
Acknowledgments
(Back)
The enthusiastic contributions of the workshop participants to this
report are gratefully recognized, especially the advice and preliminary
summaries by the session chairs Alan K Soper, ISIS, Spencer Howells, ISIS,
and Sow-Hsin Chen (MIT).
The generous support for the workshop and this report from the IPNS
staff are gratefully acknowledged. Program development funds from ORNL
and ANL to defray the costs of the workshop are also acknowledged.